Christoph E. Albers and Lorin M. Benneker
INTRODUCTION
Reportedly, nine to fifteen percent of sports injuries affect the spinal column.1-3 The majority of these injuries are benign myofascial strains responding well to physical therapy without mid- or long-term impact on athletic performance. However, more severe injuries may occur including fractures or osseous instability, disco-ligamentous instability and neurologic compromise. Two different types of injury mechanisms are distinguished. Chronic injuries most frequently involve the lumbar spine. Repetitive low energy trauma from overuse leads to structural damage such as stress fractures or degenerative changes commonly affecting the intervertebral discs (IVD) and posterior elements of the spine. This increases the incidence of low back pain (LBP) in high level athletes with up to 30% of athletes experiencing acute LBP attributable to participation in sports.4,5 Acute injuries, in contrast, are the result of high energy trauma most frequently involving the cervical, thoracolumbar and lumbar spine. Spinal cord injury with neurologic compromise can be a devastating consequence of high energy trauma of the spine. As each athletic activity can cause unique stresses and specific injury patterns, evaluating and managing sport related injuries of the spine requires a profound knowledge of the underlying anatomic structures and biomechanical conditions, the demands of the sport and the athlete, predisposing factors and mechanisms of injury of the thoracolumbar and lumbar spine. This chapter provides a summary of injuries and other pathologic conditions of the thoracic and lumbar spine related to sports highlighting current concepts of the epidemiology, underlying causes and biomechanics, diagnosis and management of athletes.
Biomechanics of the Spine in Athletes
Understanding the anatomy and functional biomechanics of the thoracolumbar spine is crucial for the evaluation of sport related injuries. Generally, the requirements to the thoracolumbar spine during physical activity are to provide flexibility and physiological range of motion while maintaining mechanical stability to counteract excessive compression, distraction, torsion or shearing forces. Acute or chronic (repetitive) extrinsic forces surpassing intrinsic stability may result in injury of the spine and its surrounding elements. Subdividing the spinal column in functional spinal units (FSU) (two adjacent vertebra, IVD, facet joints and soft tissue restraints) helps to identify specific structures at risk depending on the acting forces. Each of these elements may be injured separately or in combination. Excessive energy acting on the FSU may lead to combined injury of several or all elements resulting in gross instability and increased risk for compromise of the neurological structures. During the adolescent growth period, mechanical stresses applied to the spine differ distinctly compared to those in the skeletally mature spine. With the natural increase of lumbar lordosis during the adolescent growth spurt, the risk for posterior element injuries is elevated. Additionally, as bone mineralization lacks behind linear growth, the risk for fractures particularly involving the cartilaginous end plate ring apophysis is increased.6
In the lumbar spine, the center of gravity lies anteriorly to the spinal column leading to tensile forces applied to the posterior myofascial restraints and the gluteal muscles. The anterior structures, on the other hand, such as the vertebral bodies and IVD, are exposed to compressive forces.7,8 Both the thoracic and lumbar spine exert specific features related to their anatomy and functionality. Due to the rib cage, the thoracic spine is relatively rigid. The orientation of the facet joints in the coronal plane is neutral allowing for little flexion/extension. This makes the thoracic spine susceptible to injuries from flexion/extension forces. In contrast, in the lumbar spine facet joints are orientated in the sagittal plane leading to an increased risk for injury from rotational forces. Finally, due to the specific load sharing properties of the thoracolumbar junction (transition from rigid thoracic to mobile lumbar spine), this area is particularly vulnerable to hyperflexion, rotation and shearing forces. Depending on each athletic discipline, specific loads and stresses are transmitted to the spine. For instance, repetitive rotational and extension forces executed by gymnasts or throwers may lead to stress fractures of the pars interarticularis or the pedicle (Fig. 8-1). Understanding the direction of these extrinsic forces and their kinematic affectivity on the thoracolumbar spine helps to improve the understanding of the injury’s underlying pathophysiologic mechanisms, to make the correct diagnosis and, ultimately, to indicate correct treatment for each individual condition.
Sports Related Overuse Injuries of the Thoracolumbar Spine
Chronic overuse injuries of the thoracolumbar spine are the result of repetitive low energy micro-trauma. Often, these injuries are caused by lifelong exhibition to competitive sports with recurrent motion cycles leading to chronic overuse and stress reactions. Chronic overuse injuries can involve the anterior elements of the spine such as the disc and adjacent end plates. More commonly, the posterior structures (pars interarticularis, facet joints, spinous processes) are affected. Risk factors for chronic overuse injuries include adolescent growth spurt and contract or flaccid musculature (e.g., tight hip flexors and weak abdominal muscles leading to hyperlordosis). Chronic overuse injuries of the spine, particularly spondylolysis, is the most common cause for LBP in the adolescent and young adult accounting for 50% of all LBP.
Anterior Element Injury
Disc degeneration
Degenerative processes of the intervertebral disc contribute to a major socioeconomic burden. Their etiology is multifactorial and not fully understood. An IVD is composed of the annulus fibrosus and the nucleus pulposus. The annulus fibrosus (AF) consists mainly of type 1 collagen. With its high tensile strength, it prevents instability from distractive forces of the spine and serves as a mechanical barrier for the nucleus pulposus (NP). The latter is composed of type 2 collagen and proteins with a very high content of water. Its high viscoelastic properties allow for distributing compressive forces smoothly to the endplates of the adjacent vertebral bodies ultimately decreasing peak pressures. The increasing clinical impact of patients with IVD degeneration in the last decades is mainly attributable to the aging population. However, overuse and micro-trauma during certain sports exhibiting the IVD to repetitive shearing, compression, and distraction forces may lead to annulus tears, disc desiccation, segmental instability and compression of neurologic structures. Veres et al.9 could reproduce typical radial tears of the AF in a sheep model when applying simultaneous flexion and torsion. Moreover, in a bioreactor whole organ study setup, Chan et al.10 could show that again the combination of compression and torsion results in an significant decrease of cell viability in the NP. These pathomorphological findings may explain the high rate of disc injuries and low back pain in sports such as tennis, golf, and alpine and free skiing/snowboarding where the lumbar spine is exposed to repetitive compression in combination with torsion. Annulus tears bear the risk for herniation of the nucleus pulposus (HNP). Although HNP predominantly affects individuals during the fourth to sixth decade, there is a significant increase of elite athletes that are affected as early as 20-35 years.11,12 A previous study revealed IVD involvement in 10% of young athletes with back pain.13 HNP can be a devastating condition for elite athletes potentially affecting the athlete’s entire career. A recent review article studied the rate of elite athletes returning to their prior level of competition after lumbar discectomy.14 The proportion of athletes with full return to competition level was found to range between 75–100% of the athletes with a reported recovery time of 2.8–8.7 months after discectomy. Another meta-analysis compared return to play between non-operative treatment and discectomy in elite athletes. The authors did not reveal any differences related to return to play rates; however, they did not assess time period from injury to return to play.15
Apophyseal ring fracture
The apophyseal ring ossification centers fuse with the remaining vertebral body at the age of 17 years. Similar to HPN, compressive loading of the IVD can force the nucleus pulposus through the apophyseal physis leading to breakage of the fibrocartilaginous junction between the apophyseal ring and the vertebral body. Apophyseal ring fractures or limbus vertebra are commonly associated with vigorous sports activity before skeletal maturity. They are frequently seen in gymnasts and wrestlers. Posterior involvement of the apophyseal ring with disc herniation towards the spinal canal may cause neurologic compromise. Plain films, CT or MRI reveal the avulsed fragments. Treatment of this uncommon injury is non-operative with rest, physical therapy or bracing.
Posterior Element Injury
Spondylolysis and spondylolisthesis
Spondylolysis is a pathologic condition defined as a stress reaction of the pars interarticularis. The exact etiology is not clearly defined; however, multiple factors, such as genetic factors, skeletal immaturity or anatomic variations, seem to increase the susceptibility for pars defects. Mechanically, spondylolysis results from combined flexion, extension and rotation against resistance leading to an overload of the posterior elements. It can be unilateral; however, in up 80%, it occurs bilaterally with the most common location at the level of L5 followed by L4. Affection of more cranial lumbar levels or the thoracic or cervical spine is very rare. Although spondylolysis is equally distributed between female and male subjects, higher rates of spondylolisthesis have been reported in female athletes. Spondylolysis is significantly increased in athletes with a reported incidence of 8% among athletes in contrast to an incidence of 3-7% in the general population.16 Some athletic activities are of particular risk to develop spondylolysis. Among these are throwing sports (27%), artistic gymnasts (17%) and rowing (17%).16 Although spondylolysis can be clinically silent, the active individual commonly presents with symptoms predominated by load-dependent pain, whereas pain on rest or night pain is uncommon. On examination, patients present with hyperlordosis, tenderness on palpation, pain in hyperextension and rotation (positive stork test), and a positive one-leg hyperextension test. Neurological deficits are uncommon; however, pseudoradicular pain radiation in the buttocks or legs may be present. Radiographic work up consists of a set of plain films (AP and lateral projection). Additional 45 degree oblique views can be performed to reveal a linear radiolucency in the pedicle described as the “collar of the scotty dog” configuration. Bone scans have the highest sensitivity to detect acute spondylolysis. Due to the excellent bone to soft tissue contrast properties, computed tomography (CT) performs best to delineate the extent of the lesion and its relation to the surrounding anatomy (Fig. 8-1). Both bone scan and CT can be combined in a single photon emission computed tomography (SPECT) study. MRI is superior to eliminate radiation exposure to the predominantly young patient population. Furthermore, MRI is useful to distinguish the stage of the stress reaction allowing to depict early stages without cortical disruption (hyperintensity on T2 weighted sequences) from metabolically inactive, chronic pars defects (normo-/hypointensity on T2 weighted sequences). The success of non-operative treatment was previously assessed in a meta-analysis revealing subjective freedom of symptoms in 84% athletes after 1-year follow-up independently of bony healing of the defect.17 However, spondylolysis may be a precursor of spondylolisthesis. The etiology can be congenital (dysplastic), developmental (isthmic) or acquired (degenerative, trauma, tumor, iatrogenic) according to the Wiltse Newmann classification system. Spondylolysis is the most common cause of spondylolisthesis. Approximately 15% of all individuals with pars defects develop progression to spondylolisthesis. Less than 4% of individuals affected by low grade isthmic spondylolisthesis will develop further slip progression through skeletal maturity and adulthood. Further risk factors for adolescent and adult isthmic spondylolisthesis include spina bifida occulta, Scheuermann’s disease and a high pelvic incidence. Isthmic Spondylolisthesis almost always affects the level L5/S1 (Fig. 8-2). The degree of slippage is stratified by dividing the superior endplate of the lower adjacent vertebra (i.e., S1) into four quarters. Spondyloptosis is a term that describes complete anterior translation of the superior segment over the lower adjacent vertebra (Meyerding classification system18). On clinical examination, patients typically present with a flattened lumbar lordosis, a step off at the spinous process L4/5 and pain with single limb standing and lumbar reclination. Standing lateral plain films of the lumbar spine confirm the diagnosis. Alongside the Meyerding classification system, several slip angles on the lateral radiograph have been described to quantify the degree of the lesion.19 Furthermore, the anatomic relation of the sacrum to the pelvis is determined by measuring pelvic incidence, pelvic tilt and scaral slope and their relation to lumbar lordosis. Previous studies assessing the natural course of isthmic spondylolisthesis found comparable long-term clinical results of affected compared to healthy individuals.20 In the athletic patient, treatment aims at eliminating pain and returning to an unrestricted athletic level. Different treatment modalities have been suggested. Non-operative treatment is almost always sufficient and consists of a sport restriction and physical therapy. Bracing has not been proven to improve the overall outcome but seems to accelerate the period to return to sports. Neurologic compromise and ongoing pain after 6 months of conservative treatment and meticulous exclusion of additional sources of pain indicate surgical treatment. Surgical treatment options consists of fusion of the involved segment or direct repair of the pars interarticularis fracture.
Spinous process apophysitis
Spinous process apophysitis is a pathologic condition of the lumbar spine that exclusively affects skeletally immature athletes. It results from repetitive impingement between the spinous processes during hyperextension of the lumbar spine combined with tensile stresses from the attaching soft tissues. Spinous process apophysitis is a benign, self-limiting condition responding well to physical rest and anti-inflammatory medication. The clinical picture resembles that of spondylolysis, however, marked tenderness on palpation points more towards the spinous processes as the origin of symptoms.
Bertolotti’s syndrome
Congenital sacralization of the lowest lumbar vertebra is a rare cause of back pain in athletes. This transitional anomaly between the transverse processes of L5 and the sacrum occurs as a partial or total uni- or bilateral fusion. It is usually asymptomatic; however, hyperextension activities lead to painful irritation of the pseudoarticulation. Furthermore, an increased rate of segment instability and disc degeneration at L4/5 with sciatic pain from compression of the L5 nerve root was observed with this condition.
High Energy Injuries of the Thoracolumbar Spine in Athletes
Participating or competing in full-contact or high-speed sports exposes athletes to an increased risk for acute spinal injuries. Many of these injuries involve the cervical spine and will not be discussed in this chapter. However, injuries of the thoracic and lumbar spine, and particularly of the thoracolumbar junction with more than 50% of all fractures occurring in this region, are commonly associated with high-energy trauma obtained from sport accidents. Consecutively, acute high energy trauma of the thoracic and lumbar spine can cause spinal cord injuries with devastating neurological compromise in 8.0% of all sport accidents affecting the spine (Fig. 8-3).21 Because of the precarious blood supply in the middle thoracic spine (vascular watershed area), the risk for ischemia of the medullary cord is elevated in this region. Furthermore, the narrow spinal canal enclosing the medullary cord in the thoracic spine is more susceptible to neurologic damage from protrusion of fracture fragments into the spinal canal compared to the lumbar spine. At approximately the level of L1/2 and below, the cauda equine begins decreasing the risk for neurological damage and improving the prognosis after injury of the neurological structures as compared to the thoracic spine. Generally, the principles of patient management and treatment algorithms do not differ between acute spinal injuries from sports accidents compared to accidents unrelated to sports, and they will be discussed elsewhere. However, several studies revealed distinctly different injury patterns of acute spinal injuries sustained from sports accidents compared to accidents unrelated to sports. Siebenga et al.22 assessed acute spinal injuries obtained from horse riding and revealed 78% of all injuries occurring at the thoracolumbar junction (Th11-L2). Of those fractures, 94% were compression fractures (type A fractures according to the AO comprehensive classification system23). Franz et al. reviewed 728 patients participating in snow sports (skiing and snowboarding).24 Seventy-three patients suffered severe spinal injuries (defined as spinal fractures, subluxations, dislocations or concomitant spinal cord injuries), the majority of which were related to skiing (n = 63). The most commonly involved site was the lumbar spine; however, one half of all affected subjects suffered from injuries at least two levels of the spinal column. Neurological symptoms (transient and persistent) occurred in 38% of all patients, and none of the snowboarders were affected by persistent neurological compromise. Another study by Wasden et al.25 reviewed 1142 patients who sustained injuries from skiing (n = 794) and snowboarding (n = 348). Injuries to the thoracolumbar spine occurred in 14% of snowboarders and 10% if skiers. Gertzbein et al. stratified thoracolumbar fractures of 119 skiers and snowboarders according to the AO comprehensive classification system.23,26 Of all fractures, 95% were compression fractures (type A), of which 71% made up simple compression fractures (A1.1 – A1.3) and 23% burst fractures (A3.1 and A3.2) (Fig. 8-4). Only 4.4% had distraction type fractures and 0.9% rotational fractures. Hasler et al.27 reviewed 181 patients suffering spinal and pelvic injuries following airborne sports (11 BASE-jumpers, 144 paragliders, 19 parachuters, 1 speedflyer, 4 deltagliders, 2 skysurfer). One half suffered acute spinal injuries of which 92% were A type compression fractures, followed by C type rotational fractures (5.3%) and B type distraction injuries (4.4%). Gauler et al.28 studied 41 patients with spinal cord injury after paragliding accidents. Twenty-one patients presented with an initial American Spinal Injury Association (ASIA) score of A-C and 11 patients with ASIA score D/E. Neurologic recovery was significantly improved if spinal canal occlusion was < 70% at initial presentation and 31 of all patients left the hospital ambulatory after surgical stabilization and decompression and a mean duration of rehabilitation of 116 days.
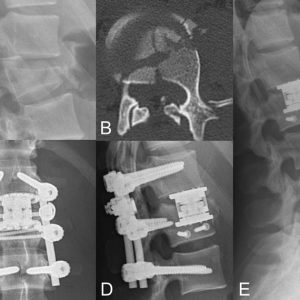
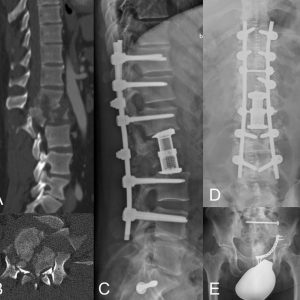
Low Back Pain in Athletes
LBP is a descriptive term for a complex of symptoms that are based on a variety of different underlying causes. LBP can have intrinsic spinal causes, but it can also originate from adjacent organs. Thus, a broad spectrum of differential diagnoses has to be assessed when evaluating patients suffering LBP. Notably, finding a structural cause for LBP is not always feasible and often the symptoms spontaneously regress hindering the treating physician to elicit the underlying pathologic condition. LBP may be caused by repetitive overuse microtrauma, acute trauma and other pathologic conditions such as inflammation, infection, deformity or tumors. Recognizing red flags necessitating further diagnostic work up is of upmost importance. The lifetime prevalence in the general population has been reported at 90 percent. Only until recently, LBP was considered rare in the adolescent and young adult. However, in the athletic population, LBP occurs in 10 to 15 percent.29 Overall duration of participation in sports, gender, and intensity and frequency of practice and performance seems to increase the incidence of LBP. It is widely accepted that the origin of LBP in the adult population differs distinctly compared to young athletic individuals. While LBP in adults is more likely due to degenerative disease such as disc pathologies, degeneration of the facet joints or degenerative stenosis, acute injuries with structural damage are more common in young athletes.13,30,31 It was previously shown that nearly 50% of athletes suffering LBP had an abnormal pars interarticularis.13,33 Furthermore, it was demonstrated that structural abnormalities of the spine vary with different types of sports. While football players suffer back pain in 50%, for instance, the prevalence of LBP in gymnasts has been reported in up to 86%.29,33 LBP originating from sport activities is often attributed to repetitive flexion, hyperextension and rotation. Selected sport activities expose the athletes to a higher risk for LBP than others. Among them are soccer, football, wrestling, weight lifting, dancing and rhythmic gymnastics, rowing, cricket, tennis weight lifting or ice hockey. However, attributing LBP and its underlying causes to a specific sport activity can be difficult, as many of the abnormalities are found in the asymptomatic subjects as well.
Activity-Specific Injury Patterns
There is an increasing body of evidence outlining the relationship of specific athletic activities to unique injury patterns. Chronic overuse injuries are typically seen in low energy sports exposing the spine to repetitive motion cycles with extreme ranges of motion. High velocity, contact, collision or combat sports, on the other hand, are prone to cause acute injuries of the spine.
Activities associated with chronic overuse injuries
Throwing and overhead sports consist of a coordinated cascade of kinematic events involving the lower limbs, rotation of the pelvis and the torso, the scapulothoracic, shoulder, elbow, wrist and finger articulations. The main role of the thoracolumbar spine is to provide static stability and level foundation during the throwing phase while transferring torque forces around the longitudinal body axis to the more distal segments of the kinematic chain. Soft tissue, bone and disc related pathologies of the spine may interfere with this well coordinated motion sequence. Muscular strains of the core muscles such as the rectus or oblique and transverse abdominal muscles adding to static stability occur in javelin throwers baseball and tennis players. Bone lesions associated with overhead activities include endplate injuries mainly in skeletally immature subjects and posterior element injuries (Fig. 8-1). A fivefold incidence of spondylolysis was found in baseball players34 and nearly one third of adolescent tennis players had spondylolysis diagnosed by MRI.35
Professional aesthetic athletes usually commence with their career at a young age. Gymnastics, ballet, dancing or figure skating expose their spine to extremes in terms of the acting forces, mobility in all dimensions and repetitive loading. The posterior elements of the lumbar spine are the most commonly affected site for chronic injuries in aesthetic sports. Spondylolysis and spondylolisthesis contribute to the major injury patterns with significantly increased prevalence in athletes performing aesthetic sports compared to the general population (Fig. 8-1 and 8-2).36-39
Similar to aesthetic sports, combat sports, including traditional martial arts as well as competitive combat disciplines, result in chronic injuries of the lumbar spine. Although combat sports are associated with acute injuries, the most commonly affected site is the cervical spine. However, previous studies have outlined the relationship between lumbar spondylolysis and martial arts as the lumbar spine is exhibited to an elevated risk for combined hyperextension, rotation and axial loading.34
Swimmers often suffer low back pain with chronic spinal injuries occurring in up to one third of all swimmers performing at a competitive level. The lumbar spine is susceptible to chronic overuse injuries in swimmers. Breast stroke and butterfly seem to be related to these injury patterns. Increased stress on the pars intraarticularis, for instance, during the dolphin kick with the lumbar spine in hyperextension, has been associated with spondylolysis most commonly at the level of L5. Alongside pars lesions, an increased prevalence of disc pathologies was demonstrated in swimmers compared to the general population in several previous trials.40,41 Additionally, an elevated prevalence of both functional and structural scoliosis was observed in swimmers. Functional scoliosis has been attributed to a muscular imbalance between the dominant and non-dominant side.42
Activities associated with acute injuries
Activities associated with acute spinal injuries include a variety of high impact and velocity sports such as winter sports, horse riding, collision sports, airborne sports or motor sports. Any of these sports can cause severe injury to the thoracolumbar spine with or without damage to the neurovascular structures. Skiing/snowboarding injuries commonly result from high energy forces leading to acute injuries of the thoracolumbar spine.24,26 A previous study investigated epidemiologic and injury patterns in 2569 patients with skiing and snowboarding accidents admitted to the emergency department.43 Nearly one third of all patients suffered injuries to the spine. The thoracolumbar spine was more commonly affected in skiers compared to sknowboarders.43 Alongside injuries from skiing or snowboarding, equestrian sports and their relation to spinal injuries have been extensively studied in the literature.22,44 Similar to skiing injuries, equestrian sports accidents most commonly result in acute injury of the spine. A previous study reported 14% of all injuries from horse riding to affect the spine.45 Another study reported a high prevalence of head, spine and trunk injuries in recreational equestrians, while professional riders were more susceptible to injuries involving the extremities.46 Hessler et al.47 found a prevalence of 60% of fractures of the thoracolumbar spine after falls of horses. A recent study including 503 patients who had suffered injuries from equestrian sports revealed that injuries to the thoracolumbar spine were the third most common injury pattern (10.9%) after head injuries (17.3%) and injuries to the upper extremities (15.2%).44 Collision and high contact sports such as American football, rugby, soccer or ice hockey bear a significant risk for acute injuries that can be associated with catastrophic spinal cord injury. The majority of fractures obtained from collision sports involve the cervical spine, and fractures affecting the thoracolumbar spine are rare,48,49 whereas avulsion fractures of the transverse processes have been reported to occur with higher incidence in athletes performing in collision and high contact sports.50
SUMMARY AND CONCLUSION
Sport injuries commonly affect the spinal column. Acute injuries typically result from high energy trauma and most frequently cause damage at the thoracolumbar junction. Chronic sport injuries are the result of repetitive low energy trauma leading to disc disease or injury of the posterior structures of predominantly the lumbar spine. Recognizing, managing and treating these injuries can be particularly challenging as high physical demands and expectations of athletes have to be respected.
RECOMMENDED READING
- de Jonge MC, Kramer J. Spine and sport. Semin Musculoskelet Radiol. 2014;18(3):246-264.
- Dunn IF, Proctor MR, Day AL. Lumbar spine injuries in athletes. Neurosurg Focus. 2006;21(4), E4.
- Menzer H, Gill GK, Paterson A. Thoracic spine sports-related injuries. Curr Sports Med Rep. 2015;14(1):34–40.
- Purcell L, Micheli L. Low back pain in young athletes. Sports Health. 2009;1(3):212–222.
- Li Y, Hresko MT. Lumbar spine surgery in athletes: outcomes and return-to-play criteria. Clin Sports Med. 2012;31(3):487-498.
- Micheli L, Stein J, O'Brien C, d’Hemecourt M, (Eds). Contemporary Pediatric and Adolescent Sports Medicine. Springer-Verlag New York; 2014.
REFERENCES
- Swärd L. The thoracolumbar spine in young elite athletes. Current concepts on the effects of physical training. Sports Med. 1992;13(5):357-364.
- Tall RL, DeVault W. Spinal injury in sport: epidemiologic considerations. Clin Sports Med. 1993;12(3):441-448.
- Williams JG. Biomechanical factors in spinal injuries. Br J Sports Med. 1980;14(1):14-17.
- Dreisinger TE, Nelson B. Management of back pain in athletes. Sports Med. 1996;21(4):313-320.
- Kelsey JL, White AA 3rd. Epidemiology and impact of low-back pain. Spine (Phila Pa 1976). 1980;5(2):133-142.
- Bailey DA, Wedge JH, McCulloch RG, Martin AD, Bernhardson SC. Epidemiology of fractures of the distal end of the radius in children as associated with growth. J Bone Joint Surg Am. 1989;71(8):1225-1231.
- Farfan HF. The biomechanical advantage of lordosis and hip extension for upright activity. Man as compared with other anthropoids. Spine (Phila Pa 1976). 1978;3(4):336-342.
- Farfan HF. Muscular mechanism of the lumbar spine and the position of power and efficiency. Orthop Clin North Am. 1975;6(1):135-144.
- Veres SP, Robertson PA, Broom ND. The influence of torsion on disc herniation when combined with flexion. Eur Spine J. 2010;19(9):1468-1478.
- Chan SC, Walser J, Ferguson SJ, Gantenbein B. Duration-dependent influence of dynamic torsion on the intervertebral disc: an intact disc organ culture study. Eur Spine J. 2015;24(11):2402-2410.
- Ong A, Anderson J, Roche J. A pilot study of the prevalence of lumbar disc degeneration in elite athletes with lower back pain at the Sydney 2000 Olympic Games. Br J Sports Med. 2003;37(3):263-266.
- Webb JH, Svien HJ, Kennedy RL. Protruded lumbar intervertebral disks in children. J Am Med Assoc. 1954;154(14):1153-1154.
- Micheli LJ, Wood R. Back pain in young athletes. Significant differences from adults in causes and patterns. Arch Pediatr Adolesc Med. 1995;149(1):15-18.
- Nair R, Kahlenberg CA, Hsu WK. Outcomes of lumbar discectomy in elite athletes: the need for high-level evidence. Clin Orthop Relat Res. 2015;473(6):1971-1977.
- Overley SC, McAnany SJ, Andelman S, et al. Return to play in elite athletes after lumbar microdiscectomy: a meta-analysis. Spine (Phila Pa 1976). 2016;41(8):713-718.
- Soler T, Calderon C. The prevalence of spondylolysis in the Spanish elite athlete. Am J Sports Med. 2000;28(1):57-62.
- Cohen E, Stuecker RD. Magnetic resonance imaging in diagnosis and follow-up of impending spondylolysis in children and adolescents: early treatment may prevent pars defects. J Pediatr Orthop B. 2005;14(2):63-67.
- Meyerding HW. Spondylolisthesis. Surg Gynecol Obstet. 1932;54:371-377.
- Lovell WW, Winter RB, Morrissy RT, et al. Lovell & Winter's Pediatric Orthopaedics. Lippincott Williams & Wilkins. 2006. ISBN:0781753589.
- Beutler WJ, Fredrickson BE, Murtland A, Sweeney CA, Grant WD, Baker D. The natural history of spondylolysis and spondylolisthesis: 45-year follow-up evaluation. Spine (Phila Pa 1976). 2003;28(10):1027-1035; discussion 1035.
- National Spinal Cord Injury Statistical Center. Figures at glance 2019. https://www.nscisc.uab.edu/Public/Facts%20and%20Figures%202019%20-%20Final.pdf. Accessed June 5, 2019.
- Siebenga J, Segers MJ, Elzinga MJ, Bakker FC, Haarman HJ, Patka P. Spine fractures caused by horse riding. Eur Spine J. 2006;15(4):465-471.
- Magerl F, Aebi M, Gertzbein SD, Harms J, Nazarian S. A comprehensive classification of thoracic and lumbar injuries. Eur Spine J. 1994;3(4):184-201.
- Franz T, Hasler RM, Benneker L, Zimmermann H, Siebenrock KA, Exadaktylos AK. Severe spinal injuries in alpine skiing and snowboarding: a 6-year review of a tertiary trauma centre for the Bernese Alps ski resorts, Switzerland. Br J Sports Med. 2008;42(1):55-58.
- Wasden CC, McIntosh SE, Keith DS, McCowan C. An analysis of skiing and snowboarding injuries on Utah slopes. J Trauma. 2009;67(5):1022-1026.
- Gertzbein SD, Khoury D, Bullington A, St John TA, Larson AI. Thoracic and lumbar fractures associated with skiing and snowboarding injuries according to the AO Comprehensive Classification. Am J Sports Med. 2012;40(8):1750-1754.
- Hasler RM, Hüttner HE, Keel MJ, et al. Spinal and pelvic injuries in airborne sports: a retrospective analysis from a major Swiss trauma centre. Injury. 2012;43(4):440-445.
- Gauler R, Moulin P, Koch HG, et al. Paragliding accidents with spinal cord injury: 10 years' experience at a single institution. Spine (Phila Pa 1976). 2006;31(10):1125-1130.
- d'Hemecourt PA, Gerbino PG 2nd, Micheli LJ. Back injuries in the young athlete. Clin Sports Med. 2000;19(4):663-679.
- 28. Kraft DE. Low back pain in the adolescent athlete. Pediatr Clin North Am. 2002;49(3):643-653.
- Purcell L, Micheli L. Low back pain in young athletes. Sports Health. 2009;1(3):212-222.
- Jackson DW. Low back pain in young athletes: evaluation of stress reaction and discogenic problems. Am J Sports Med. 1979;7(6):364-366.
- Hutchinson MR. Low back pain in elite rhythmic gymnasts. Med Sci Sports Exerc. 1999;31(11):1686-1688.
- Sakai T, Sairyo K, Suzue N, Kosaka H, Yasui N. Incidence and etiology of lumbar spondylolysis: review of the literature. J Orthop Sci. 2010;15(3):281-288.
- Alyas F, Turner M, Connell D. MRI findings in the lumbar spines of asymptomatic, adolescent, elite tennis players. Br J Sports Med. 2007;41(11):836-841; discussion 841.
- Hall SJ. Mechanical contribution to lumbar stress injuries in female gymnasts. Med Sci Sports Exerc. 1986;18(6):599-602.
- Ciullo JV, Jackson DW. Pars interarticularis stress reaction, spondylolysis, and spondylolisthesis in gymnasts. Clin Sports Med. 1985;4(1):95-110.
- Garrick JG, Requa RK. Epidemiology of women's gymnastics injuries. Am J Sports Med. 1980;8(4):261-264.
- Omey ML, Micheli LJ, Gerbino PG 2nd. Idiopathic scoliosis and spondylolysis in the female athlete. Tips for treatment. Clin Orthop Relat Res. 2000(372):74-84.
- Hangai M, Kaneoka K, Kuno S, et al. Factors associated with lumbar intervertebral disc degeneration in the elderly. Spine J. 2008;8(5):732-740.
- Kaneoka K, Shimizu K, Hangai M, et al. Lumbar intervertebral disk degeneration in elite competitive swimmers: a case control study. Am J Sports Med. 2007;35(8):1341-1345.
- Pollard H, Fernandez M. Spinal musculoskeletal injuries associated with swimming: a discussion of technique. Australas Chiropr Osteopathy. 2004;12(2):72-80.
- de Roulet A, Inaba K, Strumwasser A, et al. Severe injuries associated with skiing and snowboarding: a national trauma data bank study. J Trauma Acute Care Surg. 2017;82(4):781-786.
- Schröter C, Schulte-Sutum A, Zeckey C, Winkelmann M, Krettek C, Mommsen P. [Accidents in equestrian sports : Analysis of injury mechanisms and patterns]. Unfallchirurg. 2017;120(2):129-138.
- Hasler RM, Gyssler L, Benneker L, et al. Protective and risk factors in amateur equestrians and description of injury patterns: A retrospective data analysis and a case - control survey. J Trauma Manag Outcomes. 2011;5:4.
- Lim J, Puttaswamy V, Gizzi M, Christie L, Croker W, Crowe P. Pattern of equestrian injuries presenting to a Sydney teaching hospital. ANZ J Surg. 2003;73(8):567-571.
- Hessler C, Namislo V, Kammler G, Lockemann U, Püschel K, Meenen NM. [Spine injuries due to horse riding accidents - an analysis of 30 cases]. Sportverletz Sportschaden. 2011;25(2):93-96.
- Walsh AJ, Shine S, McManus F. Paraplegia secondary to fracture-subluxation of the thoracic spine sustained playing rugby union football. Br J Sports Med. 2004;38(6):e32.
- Magill P, Quinlan J, Kelly I. Spinal fracture in an under-age rugby player. Ir Med J. 2012;105(9):315.
- Tewes DP, Fischer DA, Quick DC, Zamberletti F, Powell J. Lumbar transverse process fractures in professional football players. Am J Sports Med. 1995;23(4):507-509.