Naresh Kumar, Shashidhar B. Kantharajanna, and Hee-Kit Wong
FACET JOINT DENERVATION
The facet joints, also known as apophyseal or zygapophyseal joints, are formed by the superior and inferior articular processes of two contiguous vertebrae. The nerves that innervate these joints can get inflamed or impinged leading to pain from the facet joints. Facet joint denervation is a procedure used to treat facet arthropathy by deactivating the nerves in the facet joints so that they can no longer transmit pain response to the brain. This is an acceptable treatment to achieve pain relief when it has been suitably determined that the pain is chronic and seems to be originating from the facet joint.
Facet Joint Innervation1,2 (Figs. 3-1 and 3-2)
The facet joint in the cervical spine is innervated by the medial branch of the dorsal ramus of the nerve exiting at the same level and the medial branch of the nerve one level below, while in the lumbar spine it is innervated by the medial branch of the dorsal ramus of the nerve exiting at the same level and the medial branch of the nerve one level above. This is due to the existence of the C8 nerve but no corresponding vertebrae. Hence, the C6-C7 facet joint is innervated by the medial branches of C6 and C7. However, the C7-T1 facet joint is innervated by the medial branches of C7 and C8. The facet joints of T1-T2 are innervated by the medial branches of C8 and T1. This pattern continues in the lumbar spine also: for instance, the L4-L5 facet joint is supplied by the medial branches originating from the L3 and L4 nerves, wherein the medial branch from the L3 nerve supplies the inferior articular process of the L4 vertebrae and the medial branch of the L4 nerve supplies the superior articular process of the L5 vertebrae. All the medial branches pass on a groove along the posterior surface of the transverse process. The innervation of L5-S1 facet joint differs from the other lumbar joints since it is innervated by the medial branch of L4 and the dorsal ramus of L5. The course of the L5 medial branch is somewhat modified because the transverse process is replaced by the ala of the sacrum. The L5 medial branch is not the medial branch of L5 but rather the dorsal primary ramus of L5, which is longer.
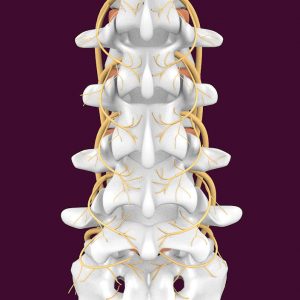
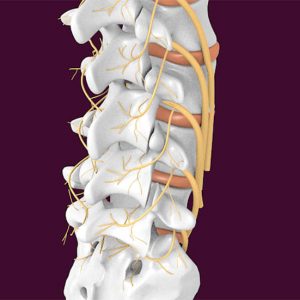
Clinical Presentation
Disorders of the facet joints present as chronic neck pain and/or chronic back pain. For cervical facet joints,3 the symptoms include neck pain, radiating pain across the nape of the neck and shoulders, and symptoms worsening with turning the head from side to side or looking up. The C2-3 and C5-6 facet joints are commonly affected segments in cases of cervical facet-mediated pain. Lumbar facet joint4 involvement presents as axial back pain or pain radiating into the buttocks up to the posterior thigh, with increased pain while standing or bending backward. L4-L5 and L5-S1 segments are commonly attributed to be the source of the pain.
The role of using confirmatory blocks before facet joint denervation remains controversial.5 All the guidelines and commissioned position papers endorsed by major spine and interventional pain societies recommend using double-level blocks to screen patients for facet joint denervation. On the other hand, Cohen et al.,5 in a multicenter analysis, concluded that the degree of pain relief obtained after diagnostic screening blocks does not correlate with zygapophyseal joint denervation outcomes. The authors further suggest proceeding to denervation procedures without diagnostic blocks. Diagnostic blocks of a facet or zygapophyseal joint can be performed by anesthetizing the joint with injections of local anesthetic/steroid intra-articularly or peri-articularly on the medial branches of the dorsal rami that innervate the joint, to evaluate whether the facet joint is the source of pain. Once the facet joint has been proven to be the source of pain, various methods are available for therapeutic facet denervation which include radiofrequency denervation (non-pulsed, pulsed, or cooled), chemical facet neurolysis, laser facet thermal ablation (also known as laser facet neurolysis), and cryodenervation.6
Intra-articular Blocks Versus “Medial Branch Blocks”7
Intra-articular injections are often more difficult and time consuming than medial branch blocks (MBBs) because they require access to the joint space, which may be extremely difficult or impossible in a degenerated facet joint. In addition, care must be taken so that injection does not rupture or overly distend the joint. The medial branch block, in contrast, requires anesthetizing both medial branches that innervate the target joint. Significant leakage of intra-articular injected fluid into the epidural space and spill over to the nerve roots have been described, which reduces the diagnostic accuracy of facet blocks. With appropriate care, this spillage is minimized with the MBB, improving its precision. Finally, intra-articular blocks are appropriate if intra-articular therapy is proposed; but if radiofrequency therapy is proposed, MBBs become the diagnostic procedure of choice. The diagnostic and therapeutic results between the two techniques (i.e., intra-articular vs. MBB) have been found to be comparable in systematic reviews; both are associated with significant rates of false-positives and negatives.8 While the false-negative response rate of MBBs has been reported to be 11%, the false-positive rate reported varies between 25% and 45%. Most investigators believe that a positive response with at least 50% improvement in clinical symptoms should be documented compared to baseline. Other investigators prefer a response of at least 80% improvement.
Technique for Intra-articular Blocks9
This procedure is done as a day care surgery procedure. The patient is placed prone on the fluoroscopy table, with a pillow under the abdomen to reduce the lumbar lordosis. The overlying skin is prepared and the C-arm is rotated until the facet joint space is first seen. This renders the beam parallel to the posterolateral part of the joint, which is accessible for direct puncture. A 22-gauge spinal needle (3½ or 5 inches) is placed on the skin so that the tip is projected over the inferior part of the joint. Using the needle tip as a marker, 5–10 mL of 1% lignocaine is injected into the skin and subcutaneous tissue. Care is taken to keep the hypodermic needle and syringe parallel to the X-ray beam. The syringe is disengaged, leaving the needle to act as a guide for the entry point and direction of the spinal needle. The latter is then introduced parallel and as close as possible to the hypodermic needle, aiming at the inferior recess of the joint, until passage through the capsule is felt and a bony end point is reached. In case of technical difficulty in entering the joint, the superior recess of the joint is the second preferred site for needle insertion. Joint entry is confirmed by instillation of 0.1–0.2 mL of the nonionic contrast agent (i.e., Iohexol), which collects in the superior and inferior recesses of the joint, giving a characteristic appearance of a seahorse on the fluoroscopy image (“seahorse sign”).9 Subsequently, 2 mL of 0.5% bupivacaine is injected for diagnostic purposes or 1 mL of 0.5% bupivacaine and 1 mL of triamcinolone for therapeutic purposes.
Technique of Medial Branch Block10
The procedure is done in a day care surgery setting and light sedation may be used. The patient is placed in a prone position. A local anesthetic is injected into the region prior to the procedure. Initially an antero-posterior view is used and the image intensifier is rotated obliquely through 15° in order to visualize the target point of medial branch nerves, the target being the “eye” of the “Scottie dog”. The spinal needle is inserted appropriately away from midline and directed obliquely down the X-ray beam. At levels L1 to L4 the medial branch block is done by targeting the junction of the upper border of the transverse process and the superior articular process. This is done at two levels for each joint in question (e.g., for the L4-L5 joint, the junction of the superior articular process and transverse process of L4 and L5 would be the target). The L5 posterior primary ramus is blocked in the groove between the ala of the sacrum and superior articular process of S1. For completeness, if the L5-S1 joint is the target, the block should be performed at the transverse process of L5, the junction of the ala of the sacrum and the superior articular process of S1, and the S1 nerve itself.
Facet Denervation
Facet denervation is a procedure by which a discrete lesion is created on the medial branches of the dorsal rami that innervate a facet joint. Although multiple techniques for denervation have been described, radiofrequency thermal-mediated ablation of the medial branch is the mainstay of facet denervation techniques.
Types of Facet Joint Denervation Available
Laser Facet Neurolysis:11 A small incision is made over the painful facet joint and, under fluoroscopy, a small tube is inserted through which a thin wire is passed to locate the nerve causing the pain. The wire is then removed and a small laser device is inserted in its place. The laser is used to debride and denervate the joint. There is a lack of published scientific evidence to support the safety and efficacy of this technique.
Percutaneous Cryodenervation:12 It is also known as cryoablation or cryoneurolysis. Under local anesthesia, a slim, luminated, double-walled cryodenervation probe, which is cooled by carbon dioxide, is brought to the location of pain to freeze the nerves and achieve a prolonged but reversible nerve facet denervation treatment.
Percutaneous Radiofrequency Facet Denervation: Also known as radiofrequency neurolysis, radiofrequency ablation (RFA), facet neurotomy, facet rhizotomy, or articular rhizolysis. Percutaneous radiofrequency facet denervation is a radiofrequency technique that uses electrodes introduced through the skin (using a local anesthetic and fluoroscopic guidance) to destroy selected nerve fibers, thereby blocking pain transmission through the neural pathways. The evidence is fair for cervical radiofrequency neurotomy. The evidence for lumbar conventional radiofrequency neurotomy is good and limited for pulsed radiofrequency neurotomy. Types of radiofrequency facet denervation used to treat chronic neck pain and/or chronic back pain include non-pulsed radiofrequency facet denervation, pulsed radiofrequency facet denervation, and cooled radiofrequency ablation.
1. Non-Pulsed Radiofrequency Facet Denervation/Conventional Radiofrequency Ablation:13
During conventional RFA an electrode introduced through the skin delivers continuous heat (produced by radio waves) to the medial branch of the ganglion that innervates the targeted facet joint at temperatures of 70–90 degrees Celsius. The intent is to damage specific nerves and interfere with their ability to transmit pain signals. Conventional RF electrodes typically create smaller lesions that do not extend much past the tip. The goal of the treatment is long-term pain relief; however, further nerve regeneration can affect the longevity of the treatment outcome, thereby requiring repeat procedures.
2. Pulsed Radiofrequency Facet Denervation/Pulsed Radiofrequency Ablation:14
Pulsed RFA has been proposed as an alternative to conventional RFA, as the former delivers intermittent bursts of heat-generated radiofrequency instead of a continuous current. During pulsed radiofrequency, short bursts of high voltage electrical current are used at temperatures that do not exceed 42 degrees Celsius, thereby reducing the risk of destruction of neighboring tissues. This technique is proposed as a possibly safer approach to non-pulsed facet denervation because it does not heat the tissue enough to cause coagulation. Both pulsed RFA and cooled RFA enable larger lesions to be treated at the treatment site than with conventional RFA, which may address the difficulty of locating lateral branch nerves during conventional RFA. The published scientific evidence is insufficient to support the efficacy and safety of pulsed radiofrequency facet denervation for the treatment of chronic pain associated with facet disease.
3. Cooled Radiofrequency Ablation:15
Cooled RFA has been proposed as another alternative to conventional RFA.13 Both pulsed RFA and cooled RFA enable larger lesions to be treated at the treatment site than those with conventional RFA, which may address the difficulty of locating lateral branch nerves during conventional RFA. Cooled RFA uses a cooling probe technology that allows for adjacent tissues to be cooled during the procedure rather than charring the tissues. Cooling is regulated by an attached computer. Internally cooled electrodes can create lesions 8 to 10 millimeters (mm) in diameter, with the depth extending distal to the electrode tip. During the cooled RFA procedure, power may be applied for longer periods of up to 150 seconds at 60 degrees Celsius or 75 degrees Celsius.
Technique of Radiofrequency Ablation
Typically, the procedure is done in an outpatient setting and light sedation may be used. The patient is placed in a prone position. A local anesthetic is injected into the region prior to the procedure and needles are introduced, positioned at the target area using fluoroscopic guidance. Continuous (non-pulsed) radiofrequency energy is targeted to the nerves using an electrode for 40 to 90 seconds at a temperature of 70–90 degrees Celsius. After the electrode is withdrawn, a small sterile dressing is applied to the site. The patient may ambulate immediately and is discharged the same day. Analgesics and anti-inflammatory medications are prescribed to ease discomfort from the denervation procedure.
INTRADISCAL ELECTROTHERMAL THERAPY
Intradiscal Electrothermal Therapy
A new method for the treatment of chronic discogenic low back pain using intradiscal electrothermal therapy (IDET) was introduced by Saal and Saal in the year 2000.16 Heat applied to the posterior annulus of the disk, with consequent contraction of collagen fibers and destruction of afferent nociceptors, is proposed to relieve the discogenic pain. Discogenic pain is deemed to arise from irritation of the small nerve endings within the annulus fibrosis. In an intradiscal electrothermal therapy procedure, radiofrequency energy is converted into heat via a thermal resistive coil contained in a flexible catheter that is percutaneously placed into the disc under fluoroscopic guidance. Heat is thus delivered at a specific temperature for a specific length of time, which thermocoagulates the nerves.
The success of the procedure depends on patient selection.17 A patient with predominant axial back pain who has failed conservative treatment over 6 weeks, with MRI showing a posterior annular tear, with (> 50%) maintained disc height and a contained disk herniation, is an ideal candidate in whom a favorable outcome can be predicted. Discogenic pain confirmed by provocation discography adds to the predictability of a favorable outcome. It is also important to rule out major facet arthropathy and psychological issues. The pain recovery after IDET is gradual. During the initial week, there might be an aggravation of pain followed by significant pain relief over 8–12 weeks. The healing process reaches its peak 4 months after the procedure. This clinical pattern of pain relief, with respect to time, arises from the fact that the disc restructuring with repair of annular tissue is time bound, with improvement in structural integrity of the disc.
Maurer et al.,18 in a prospective study, concluded that durable clinical improvements can be realized after IDET in carefully-selected patients with mild disc degeneration, confirmatory imaging evidence of annular disruption, and concordant pain provocation by low-pressure discography (defined as < 22 psi).19 However, Freeman et al.20 in an RCT—IDET compared with a sham treatment (placebo)—found that although the IDET procedure appeared to be safe with no permanent complications, no significant change in outcome measures in either group at 6 months was observed. Helm et al.,21 in a systemic review, found the evidence to be fair for IDET, using current criteria for considering successful outcomes for a treatment modality. On the other hand, recently Lu et al.22 concluded that there is insufficient evidence from randomized trials to reliably evaluate IDET and coblation nucleoplasty. They further highlighted that available evidence is based on conflicting trials, sparse and lower quality data, or non-randomized trials. The complications described in literature23 include: catheter breakage, nerve injury (cauda equina lesion), post-IDET spinal disc herniation, discitis, local infection and epidural abscess.
Radiofrequency Ablation
Radiofrequency ablation works by the principle of thermocoagulation of the nuclear material and the neural tissue supplying the annulus fibrosus.24 It also alters the constitution of the chemical mediator in the disc.24 The thermal energy in radiofrequency ablation is provided through an electrode tip creating a well-controlled spherical lesion around it. Although radiofrequency lesioning can provide temperature sufficient to thermocoagulate nervous tissue, it is limited by the distance it can transmit heat. Sometimes classified as a variant of IDET, “percutaneous intradiscal radiofrequency therapy” (PIRFT)25 is a procedure involving the placement of an electrode into the intervertebral disc and using it to apply alternating radiofrequency current. With PIRFT the radiofrequency probe is placed into the center of the disc, and the device is activated for only 90 seconds at a temperature of 70 degrees centigrade. Lu et al., in a review of RCTs and systemic review, found fair evidence that PIRFT thermocoagulation is not effective.22
Nucleoplasty
Nucleoplasty, also known as percutaneous lumbar disc decompression, is a minimally invasive technique that was first approved by the US Food and Drug Administration in 2000. It aims to achieve percutaneous disc decompression through patented coblation technology,26 in which a high-energy plasma field is generated using radiofrequency energy, through a bipolar probe. This plasma field shrinks the nucleus pulposus tissue. Two different mechanisms of action exist according to the catheter direction. The forward direction of the probe uses the coblation principle while the reverse direction uses the thermal energy principle. When the catheter moves forward, the coblation acts by producing a high-energy ionized plasma field 1 mm around the probe that breaks down the molecular bonds and dissolves the soft tissue material of the nucleus. When the catheter moves backward, coagulation occurs, created by relatively low temperatures (typically 50 °C to 70 °C) at the tip of the catheter. This process results in the shrinking of the type 2 collagen fibers present in the disc. There is weak evidence that nucleoplasty is effective in the treatment of radicular leg pain due to contained disc herniation. However, there is insufficient evidence available with regards to its role in managing discogenic, axial back pain. In the author’s personal experience,24 30 carefully-selected patients with discogenic, axial low back pain responded well to nucleoplasty for pain relief over the follow-up period of 2 years. This supports that nucleoplasty may have a role in management of discogenic axial low back pain in carefully selected patients. Cuellar et al.,27 in an observational cohort study, failed to detect any morphologic improvement of disc abnormalities by MRI evaluation in patients with persistent pain who underwent nucleoplasty. Thirty-two percent of patients in this study showed progressive degeneration in < 1 year after nucleoplasty – a rate greater than that expected by natural progression during the interval of examination. Potential complications of nucleoplasty in general include: infection, discitis, probe tip breakage or damage, bleeding, nerve damage, worsened pain, failure of technique, recurrence of herniation, paralysis, anaphylaxis and death.28
Technique of nucleoplasty
The procedure is done in day care setting with light sedation. The patient is placed in a prone position. A local anesthetic is injected into the region prior to the procedure. A 16–18G needle is introduced and positioned at the target area using fluoroscopic guidance. Using this needle, discography with contrast medium is performed to assess annular integrity. Annular integrity is confirmed by non-passage of the contrast into the epidural space. The needle is then exchanged for the access cannula using the Seldinger technique. It is customary to penetrate the disc from the more symptomatic side. The nucleoplasty wand is placed into the access cannula and advanced until the tip of the catheter is approximately 5 mm beyond the tip of the spinal needle and the access cannula is stabilized to the skin. From then on, the wand is advanced until it comes into contact with the annulus on the contralateral side. Disc decompression is done by advancing the catheter, in ablation mode, at a speed of 0.5 cm/s and withdrawing the wand in coagulation mode at a speed of 0.5 cm/s. Five to six such movements are usually made by rotating the wand in five to six different directions.
Disc Fx
Disc Fx (Elliquence, LLC) system uses manual debulking of the disc, annular modulation, and nucleus ablation while keeping the procedure minimally invasive.29 It has four steps: discography, manual discectomy, nucleus ablation, and annulus modulation. The steerable delivery system permits targeted application in the region of the pathology. High and specific ablation rate with targeted modulation of the annulus leads to a significant shrinkage by a minimum temperature distribution. Modulation of the dorsal annulus by the trigger flex probe with cauterization of inflamed structures results in shrinkage of the collagen leading to annulus shrinkage by 30%, widening of the epidural space by 10%, and resultant improvement of blood circulation and venous congestion in the epidural space. Annulus modulation may also cause reduction in pain generated by the annular nerve fibers in degenerative disc disease. Manual removal of the disc material by disc rongeurs allows adequate nerve root decompression. In carefully selected patients with sustained contained disc herniations who have failed conservative treatments, manual decompression combined with radiofrequency-assisted decompression and annulus modulation by Disc Fx was found to have good outcomes up to 4 years post-treatment.30,31
Technique of Disc Fx32
The procedure is done in day care setting with light sedation. The patient is placed in a prone position. A local anesthetic is injected into the region prior to the procedure. The side of the pathology is the preferred side of approach. The image intensifier projections of the disc and lateral border of the pedicle on an AP image are marked on the skin. Similarly, on the lateral view, the projections of the disc space and the posterior vertebral body are marked. The entry point for a 45° trajectory is about a palm’s breadth away from the midline in the AP projection of the disc space. Similarly, a horizontal trajectory will start in line with the posterior border of the relevant disc in the lateral projection. If the distance between the horizontal trajectory starting point and the midline is divided into thirds it would mark the entry points for 30° and 60° trajectories. The gap between the 30° trajectory and the zero-degree trajectory is again divided into 3 parts to get the 10° and 20° trajectory. The trajectory of the needle depends upon the location of the pathology on the preoperative MRI scan. A 20°–30° trajectory from the horizontal plane is commonly used if feasible (at L5-S1, horizontal trajectory is not possible due to the iliac crest, thereby posing the need for the trajectory to be modified). The point of entry into the disc is the postero-lateral corner as guided by the AP and lateral fluoroscopic projections. Using the above entry point and target point, a 16G or 18G spinal needle is guided lateral to the superior articular pillar (SAP), yet medial to the exiting nerve root.
After the needle is positioned appropriately, a discogram is performed using a 2 ml mixture of solution (10 ml solution = 5 ml of Iohexol dye, 3 ml of saline & 2 ml of indigo carmine). Concordance and severity of the pain and a grading of the disc morphology needs to be documented. A guide wire is then placed through the needle into the nucleus pulposus, right up to the middle of the disc on AP and lateral. A small skin incision of 3 mm is made to accommodate the introduction of the access cannula and the soft tissue dilator into the annulus. Manual discectomy of the intra-annular or sub-ligamentary disc material is done using a small disc forceps or disc rongeurs. Then the steerable Trigger-Flex (Elliquence) probe is inserted through the cannula and nucleus ablation is performed with the Surgi-Max (Elliquence) generator set at Bipolar-Turbo mode. Six strokes of ablation are carried out for the nucleus in six different directions. Then extraction of any nuclear material is done using disc irrigation. Modulation of dorsal annulus is then carried out by placing the Trigger-Flex probe under the pathological annulus and using the Bipolar-Hemo mode of the Surgi-Max generator. Four strokes of modulation are performed in different directions on the annulus, thereby covering the width of the annulus.
INTRADISCAL STEROID AND CELLULAR INJECTIONS
Intervertebral disc degeneration is a well-studied condition, yet the intricate processes involved in it are still not fully understood.33 The primary pathology of disc degeneration is initiated by painful fissuring of the annulus and some degree of nuclear degeneration. Once nuclear degeneration predominates, mechanical disc integrity is compromised which then serves as a precursor for additional disc injury. The change in disc morphology is often accompanied by altered spine biomechanics which may lead to neural irritation and degeneration of nearby tissues. It presents as a continuum of a degenerative cascade process where one form of disc pathology predisposes the patient to another. Various discs in the same person could be in different stages of this degenerative cascade.33 The three ultimate goals of interventional treatment for discogenic pain are pain relief, improved disc microenvironment, and tissue regeneration. Most of the available interventional procedures are able to achieve satisfactory results, but there are instances where pain relief may not be predictable. In such cases, the goals of treatment may be improvement in microenvironment and tissue regeneration. Intradiscal steroid and cellular injections have recently generated considerable clinical and experimental interest for their potential to alter disc microenvironment, to reduce catabolism and to regenerate disc tissue.
Intradiscal Steroid Injections
Inflammation of the neural elements, either from direct chemical irritation or secondary to an autoimmune response to the nucleus pulposus, has been implicated as the primary source in discogenic pain. Hence the rationale for using intradiscal steroids is to suppress the inflammation within the disc, thereby alleviating the pain.34 In patients with MRI evidence of degenerative disc disease and a positive response to discography, two trials found no difference between intradiscal steroid and control injection (saline or local anesthetic).35,36 However, another trial found that in patients with degenerative disc disease who failed an epidural steroid injection, intradiscal steroid injection was superior to discography alone only in the subgroup of patients with inflammatory endplate changes on MRI.37 There may be a place for this method of therapy in patients who fail lumbar epidural steroid injections and/or transforaminal epidural steroid injections, but it has not yet been proven effective. The use of intradiscal steroid injections is debated because intradiscal steroids may cause necrosis of the vertebral endplate, discitis, progression of disc degeneration, and calcification of the intervertebral disc.38 A Cochrane systematic review39 and the American Pain Society40 concluded there is no convincing scientific evidence supporting the use of intradiscal steroid injections as an alternative treatment for discogenic lumbar pain.
Intradiscal Cellular Therapy
Basic science and animal studies on cell-based therapies to treat disc disease have been reported in literature, but clinical translation of such intradiscal cellular therapy is still in its infancy. The candidate cells used for intradiscal injection include nucleus pulposus cells,41 chondrocytes42 and mesenchymal stem cells (MSCs).42 Among these, bone marrow derived MSCs are most commonly used.
Animal studies
The available animal studies are quite heterogeneous in terms of the type of animal, the model used for disc degeneration, the amount of nuclear material removed, type or quantity of cell used, the type of cellular scaffold, and the method used for analysis of outcomes related to disc morphology. The ideal animal model of lumbar disc degeneration should mimic the human degenerative process in terms of cellular, matrix, and biomechanical changes. A majority of the animal studies have reported favorable radiological and histological outcomes following cell transplantation. An experimental animal model which exactly mimics human disc degeneration has not been possible to carry out due to many concerns,43,44 including: 1. Most of the animals ethically available for research are non-chondrodystrophoid animal species, i.e., animals with persistence of notochordal cells, as they are less prone to disc degeneration; 2. The inherent difficulties in measuring pain and functional outcomes in animals; 3. Most animals available are quadrupedal with inherent biomechanical differences; 4. The small disc dimensions in these animals are disproportionate to the specifications of human discs; and 5. The complex nature of human disc degeneration, its multifactorial etiologies and lengthy time-course. Although an ideal animal model is not feasible, the evidence from such animal studies demonstrate the efficacy of cell-based treatments.45
Human studies
There are only a handful of published case reports and series reporting on human clinical trials on intradiscal cellular injections. In an open label pilot study, Pettine et al.46 reported the safety and feasibility of percutaneous injection of autologous bone marrow concentrate cells in 26 patients of chronic discogenic low back pain. The authors reported pain relief and functional improvement over 1-year follow-up. The authors also showed no worsening in disc morphology during the 1-year radiologic follow-up. Improvement in both axial back pain and radicular symptoms was noted following percutaneous administration of autologous MSCs in two elderly patients with degenerative disc disease by Yoshikawa et al.47 In a study of 10 patients with chronic low back pain and degenerative disc disease treated with intradiscal administration of autologous MSCs, Orozco et al.48 reported clinical improvement in 9 of the 10 patients. Unfortunately, these studies are not sufficient to draw any meaningful conclusions regarding the usefulness of stem cells in disc degeneration. An FDA (USA) approved phase-2 randomized controlled clinical trial, investigating the percutaneous intradiscal administration of allogeneic MSCs for single level discogenic back pain in 100 patients has now completed recruitment, but the results are not yet available.49
REFERENCES
- Bogduk N, Long M. The anatomy of the so-called “articular nerves” and their relationship to facet denervation in the treatment of low-back pain. J Neurosurg. 1979;51(2):172-177.
- Masini M, Paiva WS, Araújo AS Jr. Anatomical description of the facet joint innervation and its implication in the treatment of recurrent back pain. J Neurosurg Sci. 2005;49(4):143-146; discussion 146.
- Gellhorn AC. Cervical facet-mediated pain. Phys Med Rehabil Clin N Am. 2011;22(3):447-458, viii.
- Cohen SP, Raja SN. Pathogenesis, diagnosis, and treatment of lumbar zygapophysial (facet) joint pain. Anesthesiology. 2007;106(3):591-614.
- Cohen SP, Williams KA, Kurihara C, et al. Multicenter, randomized, comparative cost-effectiveness study comparing 0, 1, and 2 diagnostic medial branch (facet joint nerve) block treatment paradigms before lumbar facet radiofrequency denervation. Anesthesiology. 2010;113(2):395-405.
- Manchikanti L, Helm S, Singh V, et al. An algorithmic approach for clinical management of chronic spinal pain. Pain Physician. 2009;12(4):E225-E264.
- Cohen SP, Moon JY, Brummett CM, White RL, Larkin TM. Medial branch blocks or intra-articular injections as a prognostic tool before lumbar facet radiofrequency denervation: a multicenter, case-control study. Reg Anesth Pain Med. 2015;40(4):376-383.
- Datta S, Lee M, Falco FJ, Bryce DA, Hayek SM. Systematic assessment of diagnostic accuracy and therapeutic utility of lumbar facet joint interventions. Pain Physician. 2009;12(2):437-460.
- Agorastides Ioannis D, Naresh Kumar. The oblique needle technique in lumbar facet joint injection. Eur J Radiol. 2001;40(3):240-243.
- Gray D, Zahid B, Warfield C. Facet block and neurolysis. In: Waldman SD, ed. Interventional Pain Management. 2nd ed. Philadelphia, PA: WB Saunders; 2001:446-483.
- Iwatsuki K, Yoshimine T, Awazu K. Alternative denervation using laser irradiation in lumbar facet syndrome. Lasers Surg Med. 2007;39(3):225-229.
- Birkenmaier C, Veihelmann A, Trouillier H, et al. Percutaneous cryodenervation of lumbar facet joints: a prospective clinical trial. Int Orthop. 2006;31(4):525-530.
- Tekin I, Mirzai H, Ok G, Erbuyun K, Vatansever D. A comparison of conventional and pulsed radiofrequency denervation in the treatment of chronic facet joint pain. Clin J Pain. 2007;23(6):524-529.
- Gallagher RM. Pulsed radiofrequency treatment: what is the evidence of its effectiveness and should it be used in clinical practice? Pain Med. 2006;7(5):408-410.
- Kapural L, Nageeb F, Kapural M, Cata JP, Narouze S, Mekhail N. Cooled radiofrequency system for the treatment of chronic pain from sacroiliitis: the first case-series. Pain Pract. 2008;8(5):348-354.
- Saal JS, Saal JA. Management of chronic discogenic low back pain with a thermal intradiscal catheter. A preliminary report. Spine. 2000;25(3):382-388.
- Manchikanti L, Datta S, Derby R, Helm S. Intradiscal electrothermal therapy treatment for back pain. Pain Manag. 2011;1(1):41-51.
- Maurer P, Block JE, Squillante D. Intradiscal electrothermal therapy (IDET) provides effective symptom relief in patients with discogenic low back pain. J Spinal Disord Tech. 2008;21(1):55-62.
- Carragee EJ, Alamin TF, Carragee JM. Low-pressure positive Discography in subjects asymptomatic of significant low back pain illness. Spine. 2006;31(5):505-509.
- Freeman BJ, Fraser RD, Cain CM, Hall DJ, Chapple DC. A randomized, double-blind, controlled trial: intradiscal electrothermal therapy versus placebo for the treatment of chronic discogenic low back pain. Spine. 2005;30(21):2369-2377; discussion 2378.
- Helm Ii S, Deer TR, Manchikanti L, et al. Effectiveness of thermal annular procedures in treating discogenic low back pain. Pain Physician. 2012;15(3):E279-304.
- Lu Y, Guzman JZ, Purmessur D, et al. Nonoperative management of discogenic back pain: a systematic review. Spine. 2014;39(16):1314-1324.
- Kallewaard JW, Terheggen MA, Groen GJ, et al. 15. Discogenic low back pain. Pain Pract. 2010;10(6):560-579.
- Kumar NS, Shah SM, Tan BW, Juned S, Yao K. Discogenic axial back pain: is there a role for nucleoplasty? Asian Spine J. 2013;7(4):314-321.
- Barendse GA, van Den Berg SG, Kessels AH, Weber WE, van Kleef M. Randomized controlled trial of percutaneous intradiscal radiofrequency thermocoagulation for chronic discogenic back pain: lack of effect from a 90-second 70 C lesion. Spine. 2001;26(3):287-292.
- Chen YC, Lee SH, Chen D. Intradiscal pressure study of percutaneous disc decompression with nucleoplasty in human cadavers. Spine. 2003;28(7):661-665.
- Cuellar VG, Cuellar JM, Vaccaro AR, Carragee EJ, Scuderi GJ. Accelerated degeneration after failed cervical lumbar nucleoplasty. J Spinal Disord Tech. 2010;23(8):521-524.
- Yan D, Li J, Zhu H, Zhang Z, Duan L. Percutaneous cervical nucleoplasty and percutaneous cervical discectomy treatments of the contained cervical disc herniation. Arch Orthop Trauma Surg. 2010; 130: 1371-1376.
- Hellinger S, Disc FX. A treatment for discal pain syndromes combining a manual and radiofrequency assisted posterolateral decompressive nucleotomy. Eur Musculoskel Review. 2011;6(2):100-104.
- Hellinger S. Treatment of contained lumbar disc herniations using radiofrequency assisted micro-tubular decompression and nucleotomy: four-year prospective study results. Int J Spine Surg. 2014;8:24.
- Park CH, Lee SH. Efficacy of nucleo-annuloplasty using Disc-Fx in lumbar disc herniation. J Spine. 2015;4:275.
- Kumar N, Kumar A, Siddharth M S, Sambhav P S, Tan J. Annulo-nucleoplasty using Disc-FX in the management of lumbar disc pathology: early results. Int J Spine Surg. 2014;8:18.
- Bogduk N, Aprill C, Derby R. Lumbar discogenic pain: state-of-the-art review. Pain Med, 2013;14(6):813-836.
- Buttermann GR. The effect of spinal steroid injections for degenerative disc disease. Spine J. 2004;4(5):495-505.
- Woodward AH. Re: Khot A, Bowditch M, Powell J, et al. The use of intradiscal steroid therapy for lumbar spinal discogenic pain: a randomized controlled trial. Spine 2004;29:833-7. Spine. 2004;29(21):2474-2475.
- Simmons JW, McMillin JN, Emery SF, Kimmich SJ. Intradiscal steroids. A prospective double-blind clinical trial. Spine. 1992;17(6 Suppl):S172-S175.
- Cao P, Jiang L, Zhuang C, et al. Intradiscal injection therapy for degenerative chronic discogenic low back pain with end plate Modic changes. Spine J. 2011;11(2):100-106.
- Berthelot JM, Le Goff B, Maugars Y. Side effects of corticosteroid injections: what's new? Joint Bone Spine. 2013;80(4):363-367.
- Staal JB, de Bie RA, de Vet HC, Hildebrandt J, Nelemans P. Injection therapy for subacute and chronic low back pain: an updated Cochrane review. Spine. 2009;34(1):49-59.
- Chou R, Atlas SJ, Stanos SP, Rosenquist RW. Nonsurgical interventional therapies for low back pain: a review of the evidence for an American Pain Society clinical practice guideline. Spine. 2009;34(10):1078-1093.
- Nomura T, Mochida J, Okuma M, Nishimura K, Sakabe K. Nucleus pulposus allograft retards intervertebral disc degeneration. Clin Orthop Relat Res. 2001;(389):94-101.
- Acosta FL Jr, Metz L, Adkisson HD, et al. Porcine intervertebral disc repair using allogeneic juvenile articular chondrocytes or mesenchymal stem cells. Tissue Eng Part A. 2011;17(23-24):3045-3055.
- Oehme D, Goldschlager T, Ghosh P, Rosenfeld JV, Jenkin G. Cell-based therapies used to treat lumbar degenerative disc disease: a systematic review of animal studies and human clinical trials. Stem Cells Int. 2015;2015:946031.
- Kumar N, Kukreti S, Ishaque M, Mulholland R. Anatomy of deer spine and its comparison to the human spine. Anat Rec. 2000;260(2):189-203.
- Meisel HJ, Siodla V, Ganey T, Minkus Y, Hutton WC, Alasevic OJ. Clinical experience in cell-based therapeutics: disc chondrocyte transplantation A treatment for degenerated or damaged intervertebral disc. Biomol Eng. 2007;24(1):5-21.
- Pettine KA, Murphy MB, Suzuki RK, Sand TT. Percutaneous injection of autologous bone marrow concentrate cells significantly reduces lumbar discogenic pain through 12 months. Stem Cells. 2015;33(1):146-156.
- Yoshikawa T, Ueda Y, Miyazaki K, Koizumi M, Takakura Y. Disc regeneration therapy using marrow mesenchymal cell transplantation: a report of two case studies. Spine. 2010;35(11):E475-E480.
- Orozco L, Soler R, Morera C, Alberca M, Sánchez A, García-Sancho J. Intervertebral disc repair by autologous mesenchymal bone marrow cells: a pilot study. Transplantation. 2011;92(7):822-828.
- NIH. Safety and Preliminary Efficacy Study of Mesenchymal Precursor Cells (MPCs) in Subjects with Chronic Discogenic Lumbar Back Pain. ClinicalTrials.Gov. 2011.